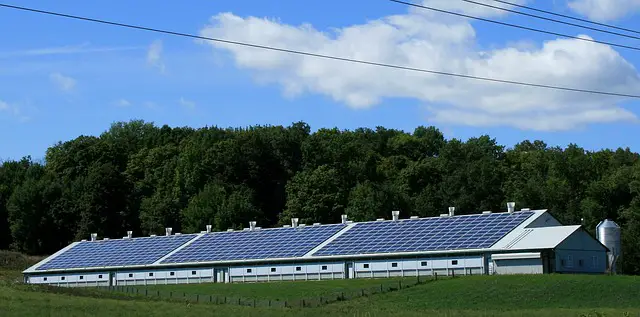
Introduction
Carbon Capture and Storage (CCS) is a process by which we can trap carbon dioxide (CO2) before it escapes into the atmosphere and store it safely. This revolutionary technology is one of the most promising solutions in our battle against climate change. By capturing CO2 from power plants and industrial sources and storing it underground, CCS can significantly reduce the amount of greenhouse gases that contribute to global warming.
The Science Behind Carbon Capture and Storage
How Does Carbon Capture and Storage Work?
CCS involves three main steps: capturing CO2, transporting it to a storage site, and storing it safely away from the atmosphere. The process begins with capturing the CO2 produced by burning fossil fuels in power plants and industrial processes. Once captured, the CO2 is compressed and transported via pipelines to a storage location where it is injected deep underground into rock formations.
Different Methods of Capturing Carbon
Carbon capture methods are technologies and techniques used to capture carbon dioxide (CO₂) emissions from industrial processes, power generation, and other sources before they enter the atmosphere. Here are some primary methods of carbon capture:
1. Pre-Combustion Capture
- Description: Involves gasifying fossil fuels to produce a synthesis gas (syngas) composed of hydrogen (H₂) and carbon monoxide (CO). The CO is then converted to CO₂, which can be captured.
- Applications: Common in integrated gasification combined cycle (IGCC) power plants.
2. Post-Combustion Capture
- Description: Captures CO₂ from the flue gases emitted after fossil fuels are burned. This method uses chemical solvents, such as amines, to absorb CO₂ from the exhaust gases.
- Applications: Retrofitting existing power plants and industrial facilities.
3. Oxy-Fuel Combustion
- Description: Burns fossil fuels in a mixture of pure oxygen and recirculated flue gas instead of air, resulting in a flue gas that is mainly water vapor and CO₂. The water vapor is condensed, leaving almost pure CO₂ for capture.
- Applications: Power plants and industrial processes where high CO₂ concentration is beneficial.
4. Direct Air Capture (DAC)
- Description: Involves capturing CO₂ directly from the ambient air using chemical sorbents. The CO₂ is then released from the sorbents through heating or chemical reactions and can be stored or utilized.
- Applications: Can be deployed anywhere, often in locations with favorable storage or utilization opportunities.
5. Bioenergy with Carbon Capture and Storage (BECCS)
- Description: Combines biomass energy production with CO₂ capture and storage. Biomass absorbs CO₂ as it grows, and when it is burned for energy, the CO₂ emissions are captured and stored, resulting in a net reduction of atmospheric CO₂.
- Applications: Biomass power plants, biofuel production facilities.
6. Chemical Looping Combustion (CLC)
- Description: Uses metal oxides as oxygen carriers to transfer oxygen from air to fuel, creating a separate CO₂ stream that can be captured. This avoids direct contact between air and fuel.
- Applications: Power generation and industrial processes.
7. Mineralization and Carbonation
- Description: Involves reacting CO₂ with naturally occurring minerals to form stable carbonates, effectively storing CO₂ in a solid form.
- Applications: Industrial waste treatment, construction materials.
8. Membrane Separation
- Description: Uses selective membranes to separate CO₂ from other gases in the flue stream. The membranes allow CO₂ to pass through while blocking other gases.
- Applications: Can be integrated into existing industrial processes and power plants.
9. Cryogenic Separation
- Description: Involves cooling the flue gases to very low temperatures to separate CO₂ by condensation and sublimation.
- Applications: Power plants and industries with high-purity CO₂ requirements.
10. Hybrid Systems
- Description: Combines multiple carbon capture technologies to enhance efficiency and effectiveness.
- Applications: Custom solutions for complex industrial processes and large-scale power generation.
Storage Locations
Captured CO2 can be stored in various geological formations, including depleted oil and gas fields, deep saline aquifers, and unmineable coal seams. These underground storage sites are chosen for their ability to securely trap CO2 for thousands of years.
History of Carbon Capture and Storage
Early Developments and Milestones
The concept of CCS has been around since the 1970s, but it wasn’t until the 21st century that it gained significant attention. Early projects, such as the Sleipner CO2 Storage Project in Norway, demonstrated the feasibility of CCS on a large scale.
Key Projects and Their Impact
Notable projects like the Boundary Dam in Canada and the Gorgon CO2 Injection Project in Australia have shown that CCS can be effectively implemented, reducing millions of tons of CO2 emissions annually.
The Benefits of Carbon Capture and Storage
Environmental Advantages
CCS plays a crucial role in mitigating climate change by significantly reducing CO2 emissions from industrial sources and power plants. This helps slow the rate of global warming and its associated impacts.
Economic Benefits
Implementing CCS can stimulate economic growth by creating new jobs in the engineering, construction, and operational sectors. It also helps industries transition to low-carbon processes, maintaining competitiveness in a carbon-constrained world.
Enhancing Energy Security
CCS allows for the continued use of fossil fuels while reducing their environmental impact. This helps ensure a stable and reliable energy supply during the transition to renewable energy sources.
Types of Carbon Capture Technologies
Pre-combustion Capture
Pre-combustion capture involves converting fossil fuels into a mixture of hydrogen and CO2 before combustion. The CO2 is then separated and captured, while the hydrogen can be used as a clean fuel.
Post-combustion Capture
Post-combustion capture is the most widely used method, involving the removal of CO2 from the flue gases after fossil fuels have been burned. This is typically done using chemical solvents that absorb CO2.
Oxy-fuel Combustion
Oxy-fuel combustion burns fossil fuels in pure oxygen, producing a flue gas that is primarily water vapor and CO2. The water vapor is condensed, leaving a high-purity CO2 stream that can be captured.
Storage Solutions
Geological Storage
Geological storage involves injecting captured CO2 into deep underground rock formations. These formations, such as depleted oil and gas fields and deep saline aquifers, can securely contain CO2 for millennia.
Ocean Storage
Ocean storage entails injecting CO2 into the deep ocean, where it can be sequestered for hundreds to thousands of years. However, this method is controversial due to potential environmental impacts on marine ecosystems.
Mineral Storage
Mineral storage, or mineral carbonation, involves reacting CO2 with naturally occurring minerals to form stable carbonates. This method offers permanent storage but is currently more costly and less developed than geological storage.
CCS and Renewable Energy Integration
How Carbon Capture and Storage Complements Renewable Energy
CCS can work alongside renewable energy sources to reduce overall greenhouse gas emissions. By capturing emissions from fossil fuel-based power plants, CCS allows for a smoother transition to a renewable-dominated energy mix.
Case Studies of Successful Integration
Several projects worldwide demonstrate successful integration of CCS with renewable energy. For instance, the Petra Nova project in the US combines CCS with enhanced oil recovery, showcasing the technology’s potential in diverse applications.
Challenges and Limitations
Technical Challenges
CCS faces several technical challenges, including the need for advanced materials and processes to capture and store CO2 effectively. Additionally, monitoring and verifying stored CO2 over long periods is complex and requires robust systems.
Economic and Financial Barriers
The high costs associated with CCS implementation pose significant economic barriers. Investment in infrastructure, operation, and maintenance is substantial, making it crucial to develop cost-effective technologies and secure funding.
Policy and Regulatory Issues
Effective policy frameworks and regulatory support are essential for CCS deployment. Governments must establish clear guidelines and incentives to encourage investment and development in CCS technologies.
Global Initiatives and Policies
International Agreements and Commitments
International agreements like the Paris Agreement recognize the importance of CCS in achieving global climate goals. Countries are increasingly committing to CCS as part of their national climate action plans.
Country-specific Policies and Projects
Countries like the US, Canada, Norway, and Australia have developed specific policies and projects to support CCS deployment. These initiatives include funding, regulatory frameworks, and research programs.
Future Prospects of CCS
Technological Advancements on the Horizon
Ongoing research and development are driving technological advancements in CCS. Innovations in capture materials, storage methods, and monitoring technologies are expected to enhance efficiency and reduce costs.
Potential for Global Adoption
As climate change concerns intensify, the potential for global adoption of CCS grows. Collaboration between governments, industries, and research institutions is essential to scale up CCS deployment worldwide.
CCS in Action: Case Studies
Notable CCS Projects Around the World
Prominent CCS projects, such as the Sleipner Project in Norway, the Quest Project in Canada, and the Gorgon Project in Australia, highlight the diverse applications and successes of CCS technology.